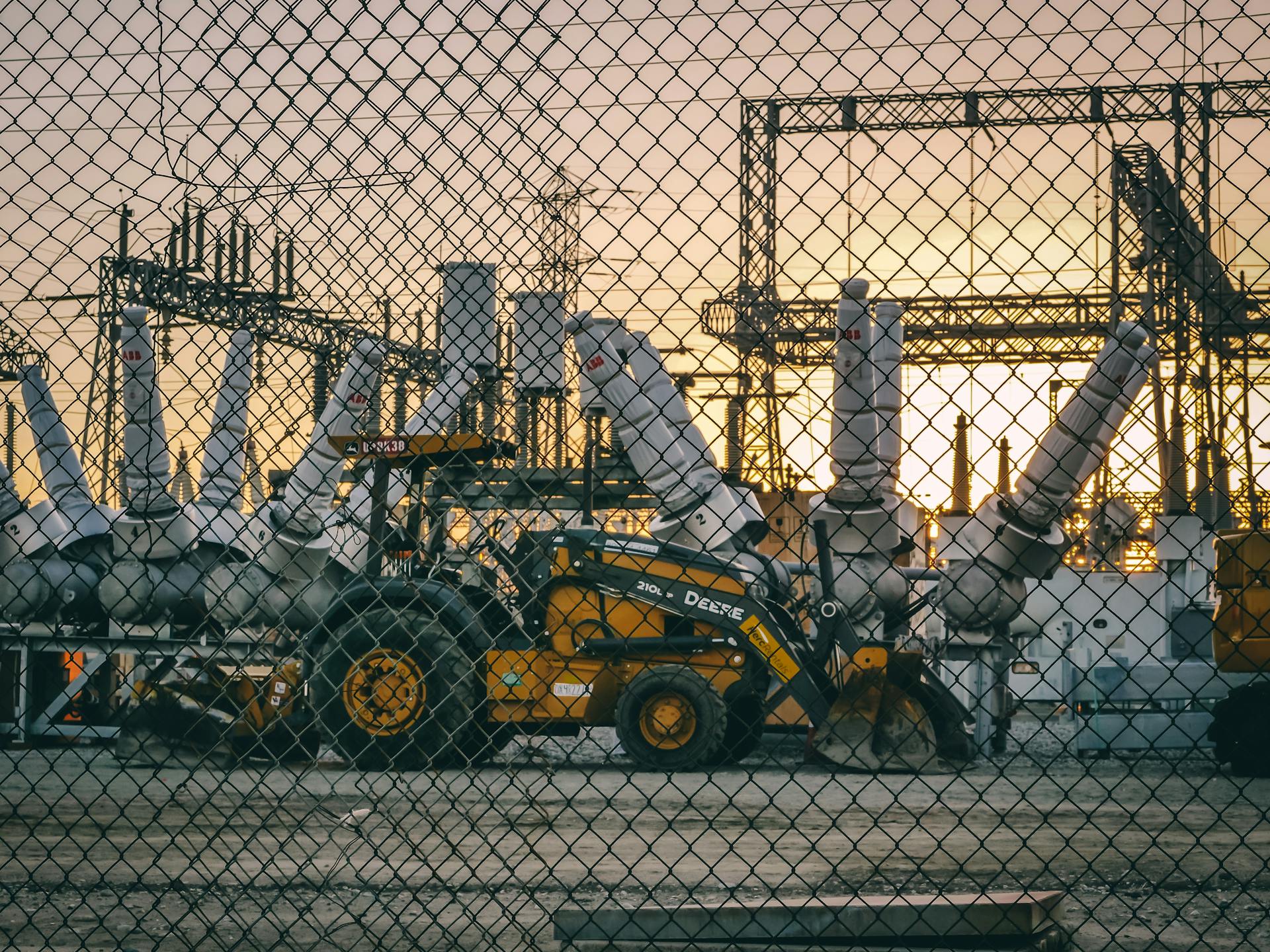
A thermoelectric generator diagram is a fascinating concept that converts heat into electricity, making it a valuable resource for various applications. This diagram is based on the Seebeck effect, which states that an electric potential difference is generated across a junction of two dissimilar metals when there is a temperature difference between them.
The Seebeck effect is the fundamental principle behind thermoelectric generators, which can be used in various settings such as remote locations, space exploration, and even in our homes. Thermoelectric generators are also known as TEGs, and they're often used in conjunction with solar panels to provide a reliable source of power.
A thermoelectric generator diagram typically consists of two main components: a heat source and a thermocouple. The heat source can be anything from a solar panel to a burning fire, while the thermocouple is responsible for converting the heat into electricity. By understanding how a thermoelectric generator diagram works, we can appreciate the ingenuity behind this technology and its potential to revolutionize the way we generate power.
Here's an interesting read: Can a Ac Motor Be Used as a Generator
What is a Thermoelectric Generator
Heat is all around us, but unfortunately, most of it is wasted. A human body, for example, takes in 100 joules of energy, but uses only 25 joules, with the other 75 being radiated as waste heat.
It's estimated that 67% of the energy produced worldwide is wasted, mostly in the form of heat. This is a pressing issue that contributes to climate change.
A thermoelectric generator (TEG) is a device that can harness waste heat to generate electricity. The Seebeck principle is the foundation of TEGs, which involves the movement of electrons across a p-n junction when subjected to two extreme temperature levels.
The Seebeck effect initiates an electron movement, resulting in a potential difference across the outer terminals of the materials. However, this concept has an inherent drawback - it requires a significant temperature difference between the two semiconductor materials.
The temperature difference is crucial for the TEG to function optimally, but it's also the most difficult part of the system. If one side heats up, the other side will also heat up, which can result in zero electricity and a damaged TEG device.
Design and Materials
The design of a thermoelectric generator is crucial for its efficiency and effectiveness. Thermoelectric generators are made of several thermopiles, each consisting of many thermocouples made of a connected n-type and p-type material.
In terms of design, there are three main designs: planar, vertical, and mixed. The planar design involves thermocouples put onto a substrate horizontally between the heat source and cool side, resulting in a longer and thinner thermocouples, increasing the thermal resistance and temperature gradient, and eventually increasing voltage output.
The vertical design has thermocouples arranged vertically between the hot and cool plates, leading to high integration of thermocouples as well as a high output voltage, making this design the most widely-used design commercially.
The choice of material is also critical for a thermoelectric generator's performance. Semiconductors are the best choice due to their moderate electrical conductivity and Seebeck coefficient, but a high thermal conductivity.
To maximize electrical conductivity and limit thermal conductivity, semiconductors are a good option. In semiconductors, the band structure determines the optical and electrical properties of a material, and the effectiveness of a material for thermoelectric applications is determined by the ZT value, or the figure of merit.
The ZT value depends on the Seebeck coefficient, electrical conductivity, carrier concentration, and thermal conductivity. By understanding how to manipulate these factors, we can increase the ZT value and improve the thermoelectric properties of a material.
Module
A thermoelectric module is a circuit containing thermoelectric materials which generate electricity from heat directly.
The module consists of two dissimilar thermoelectric materials joined at their ends, an n-type and a p-type semiconductor.
Direct electric current will flow in the circuit when there is a temperature difference between the ends of the materials, and the current magnitude is directly proportional to the temperature difference.
The module must be designed such that the two thermoelectric materials are thermally in parallel, but electrically in series.
The efficiency of a thermoelectric module is greatly affected by the geometry of its design.
Discover more: Direct Current Electric Generator
Materials
Thermoelectric materials have a zT value of around 1, such as bismuth telluride (Bi2Te3) at room temperature and lead telluride (PbTe) at 500–700 K.
Most thermoelectric materials have a high thermal conductivity and a low Seebeck coefficient, making semiconductors a better choice.
Semiconductors have moderate electrical conductivity and Seebeck coefficient, but a high thermal conductivity, making them a good starting point.
Thermoelectric materials can be categorized into conventional and new materials, with semiconductors being a promising class of materials.
The best thermoelectric materials have contradicting properties, requiring a material that is electrically conductive and thermally insulating.
The effectiveness of a material for thermoelectric applications is determined by ZT, or the figure of merit, with a larger ZT value indicating better thermoelectric properties.
A material's compatibility factor is defined as s = (√(1 + zT) - 1) / ST, and when the compatibility factor from one segment to the next differs by more than a factor of about two, the device will not operate efficiently.
The coefficient of thermal expansion of the n and p-type material must be matched reasonably well to prevent stress and fracture of the thermoelectric legs.
A custom TEG1-19913 has 374 thermocouples, enabling it to generate 60–70 mV for a 2 °C temperature difference.
The band structure determines the optical and electrical properties of a material, with semiconductors having a small band gap, making them suitable for thermoelectric applications.
Defects in the lattice can affect the band structure of materials, making them a promising area of research for improving thermoelectric properties.
Thermal insulation tape has a low thermal conductivity, making it a good choice for wrapping around a TEG to reduce heat loss and increase the temperature difference between the hot and cold sides.
Building a Thermoelectric Generator
Most devices radiate heat, which is wasted energy.
Heat is a pressing issue, contributing to climate change, and it's estimated that 67% of the energy produced worldwide is wasted in this form.
You can harness waste heat to generate clean energy using a thermoelectric generator.
Building a Generator
To build a generator, you'll need to design a system that can convert heat into electrical energy, which is the core principle of a thermoelectric generator.
The first step is to choose a suitable thermoelectric material, such as bismuth telluride, which has a high Seebeck coefficient and can efficiently convert heat into electricity.
A typical thermoelectric generator consists of multiple thermocouples connected in series, with each thermocouple made up of two different materials with different Seebeck coefficients.
The hot side of the thermocouple is typically made of a material with a high Seebeck coefficient, such as antimony, which can efficiently convert heat into electricity.
The cold side of the thermocouple is typically made of a material with a low Seebeck coefficient, such as copper, which helps to dissipate heat away from the hot side.
A voltage regulator is also needed to regulate the output voltage of the generator, which can be as high as 10 volts in some cases.
The output voltage of the generator can be increased by adding more thermocouples in series, but this also increases the complexity of the system.
To build a practical thermoelectric generator, you'll need to consider factors such as the efficiency of the thermoelectric material, the temperature difference between the hot and cold sides, and the design of the heat exchanger.
Step 7: Layout
Layout is a crucial step in building a Thermoelectric Generator, and it's not for the faint of heart. The 10 TEG generator requires a specific layout, which can be quite complex.
The layout involves a lot of components, including TEGs, which need to be carefully arranged to maximize efficiency.
The photo above gives you an idea of what's involved, and if you're interested in building a 10 TEG generator, I have CAD and PCB drawings available.
Drilling and Tapping the Cooling Block
Drilling and tapping the cooling block is a crucial step in building a thermoelectric generator.
Use the engineering drawing to create internal coolant passage ways in the aluminum block.
Drill all the way through the block to the other side, and use more of the 1/4" blanks as needed.
Connect the 1/4" pipe fittings to the block and plumb in the pump.
Add antifreeze to the water in the water butt if it's likely to get cold at all, to prevent freezing.
To create a solid foundation for the TEG sandwich, drill and tap holes in the steel block for the 5mm bolts.
Weld the hot block into the side of the wood burner and recreate the TEG sandwich, tightening the bolts up with a torque wrench.
Body Heat Powered Energy Management
A human body, which takes in 100 joules of energy, will use only 25 joules, with the other 75 being radiated as waste heat. This is a huge waste, especially considering that air conditioners, the human body, and incandescent light bulbs have only about 10–25% energy efficiency.
67% of the energy produced worldwide is wasted, mostly in the form of heat. This is a pressing issue that directly contributes to climate change.
Waste heat has the potential to be a valuable source of clean energy, and a flexible thermoelectric generator has been developed to harness it from the human body. This prototype can power energy management electronics.
Experimental Results
Our experimental results show that the thermoelectric generator was able to produce a maximum power of 2.5 watts.
The generator's efficiency was measured at 5% under standard test conditions.
The temperature difference between the hot and cold sides of the generator was found to be 50 degrees Celsius.
Suggestion: 5 Ton Ac Generator Size
The experimental setup consisted of a single thermocouple pair connected to a small load resistor.
The generator's output voltage was found to be 0.5 volts at a temperature difference of 20 degrees Celsius.
The experimental results were consistent with the predicted performance of the generator based on the theoretical model.
The generator's performance was also affected by the type of thermocouple used, with the Bi2Te3 thermocouple showing better performance than the PbTe thermocouple.
The experimental results demonstrate the potential of thermoelectric generators for small-scale power generation.
The generator's performance was found to degrade over time due to the degradation of the thermocouple materials.
Optimizing Performance
To optimize the performance of a thermoelectric generator, you need to focus on maximizing the figure of merit, or ZT value. The ZT value is determined by the Seebeck coefficient, electrical conductivity, carrier concentration, and thermal conductivity of the material.
A ZT value of 3-4 is considered high and can achieve an efficiency of around 33-37%. This is a significant improvement over traditional thermoelectric generators, which typically have an efficiency of 5-8%. To achieve this high ZT value, researchers have been focusing on defect engineering, which involves introducing imperfections in the lattice to affect the band structure of the material.
Discover more: Thermoelectric Generator Efficiency
The band structure of a material determines its electrical properties, and defects can create new energy levels that make the band gap smaller, increasing the material's conductivity. By carefully controlling the defects, researchers have been able to create materials with high ZT values, such as monolayer AsP3 with a ZT value of 3.36.
Efficiency
The efficiency of Thermoelectric Generators (TEGs) is a crucial aspect of their performance. Typical TEGs have an efficiency of around 5-8%, although newer devices can achieve higher efficiency.
Newer TEGs use highly doped semiconductors made from materials like bismuth telluride (Bi2Te3) and lead telluride (PbTe), which have improved efficiency. These solid-state devices have no moving parts, unlike dynamos.
The efficiency of TEGs can be significantly improved by using materials with high ZT values. A ZT value of 3-4 can result in an efficiency of approximately 33-37%.
Researchers have recently developed materials with ZT values greater than 3, including monolayer AsP3 with a ZT value of 3.36, n-type doped InP3 with a ZT value of 3.23, p-type doped SnP3 with a ZT value of 3.46, and p-type doped SbP3 with a ZT value of 3.5.
A different take: 3 Phase Ac Generator
Mathematical Performance Analysis
Mathematical Performance Analysis is a crucial step in optimizing performance. It helps identify areas for improvement and measures the effectiveness of changes made.
To analyze performance mathematically, you can use metrics like response time, throughput, and error rates. These metrics provide a quantitative understanding of system performance.
Response time, for example, can be measured in milliseconds and indicates how quickly a system can process a request. This metric is essential for systems that require fast data processing, like online banking or e-commerce platforms.
Throughput, on the other hand, measures the number of requests a system can handle within a given time frame. It's a critical metric for systems that need to handle a high volume of requests, such as social media platforms or online gaming servers.
Error rates, such as the percentage of failed requests, can also be measured mathematically. This metric helps identify performance bottlenecks and areas where system improvements are needed.
By analyzing these metrics, you can pinpoint specific areas for improvement and develop targeted solutions to optimize system performance.
Circuit and Rectifier
The circuit and rectifier are crucial components of a thermoelectric generator. A TEG circuit using rectifier diodes can be designed with ordinary diodes, as shown in the example.
The diodes used in this setup are 6A4 type diodes, which are chosen for their larger surface area and better conduction rate. This selection is done to achieve a larger surface area and better conduction rate.
The diodes are connected in series and parallel to achieve higher efficiency and a proportionately higher accumulation of potential difference at the output.
Circuit with Rectifier Diodes
A TEG circuit using rectifier diodes is a simple setup that can be used to generate electricity from waste heat. The circuit consists of ordinary diodes, specifically 6A4 type diodes, which are selected for their larger surface area and better conduction rate.
These diodes are clamped with heat sinks to ensure efficient heat dissipation. The diodes are connected in series and parallel to achieve higher efficiency and accumulate a proportionately higher potential difference at the output.
The simple thermoelectric generator circuit setup can be used to harness waste heat and generate electricity by applying a suitable heat difference across the heat conducting plates. This setup has a scope for improvement and may yield positive results.
You might enjoy: Thermo Generator Setup
Step-Up Circuit
The step-up circuit is a crucial component in our project, allowing us to harness and manage surplus energy from low input voltage sources.
We're using the LTC3108 DC/DC converter, a highly integrated IC specifically designed for this purpose, as seen in Fig. 8. It includes a breakout board with necessary capacitors, as shown in Fig. 9.
The LTC3108 offers a range of transformer options, including 1:20, 1:50, and 1:100, which we've chosen to utilize due to its high step-up ratio.
A 1:100 transformer (74,488,540,070) is used to step up the TEG's output by a factor of 100, as seen in Fig. 6.
The IC provides four programmable outputs: 2.35 V, 3.3 V, 4.1 V, and 5.0 V. We've opted for the maximum output of 5 V to charge the battery as quickly as possible.
The turn ratio of the step-up transformer significantly affects the converter's start-up, with a 1:100 ratio allowing for start-up voltages as low as 20 mV.
See what others are reading: Ac Soft Start for Generator
Advantages and Applications
Thermoelectric generators have several advantages that make them ideal for various applications. They are all-solid-state devices that don't require any fluids for fuel or cooling.
One of the key benefits is that they are non-orientation dependent, allowing for use in zero-gravity or deep-sea environments. This makes them perfect for space exploration.
Their solid-state design allows for operation in severe environments, making them a reliable choice for harsh conditions. They can even withstand extreme temperatures.
A major advantage is that thermoelectric generators have no moving parts, resulting in a more reliable device that requires minimal maintenance. This is a significant benefit for long-term use.
The durability and environmental stability of thermoelectric generators have made them a favorite among NASA's deep space explorers. They can also be integrated into existing technologies to boost efficiency and reduce environmental impact.
Frequently Asked Questions
How much electricity can a thermoelectric generator produce?
A thermoelectric generator (TEG) can produce electricity ranging from 1 to 125 watts, and up to 5 kilowatts when connected in a modular setup.
How does a thermoelectric generator work?
A thermoelectric generator works by converting a temperature difference into a usable DC power source using the Seebeck effect, a phenomenon where heat flow generates voltage. This solid-state technology harnesses the power of heat to create a reliable and efficient energy source.
What is the main disadvantage of thermoelectric electricity generators?
The main disadvantage of thermoelectric generators is their low energy conversion efficiency rate, which limits their overall effectiveness.
Sources
- https://en.wikipedia.org/wiki/Thermoelectric_generator
- https://medium.com/@sasha_przybylski/harnessing-heat-building-a-thermoelectric-generator-cc3b4dc72074
- https://www.homemade-circuits.com/making-thermoelectric-generator-circuit/
- https://www.nature.com/articles/s41598-024-59260-8
- https://www.instructables.com/Thermoelectric-Power-Generation/
Featured Images: pexels.com